Biopolymers include any polymers produced by living cells, constructed from repeating monomers that are linked together to form a longer chain. Examples of monomers include nucleic acids, sugars, fatty acids, and phosphates, which are generally assembled into biopolymers by processive enzymes. Bacteria produce biopolymers naturally to facilitate propagation, being essential to energy storage, structure creation, and biofilm generation.
Image Credit: Vink Fan/Shutterstock.com
Given the key role played by biopolymers in the pathogenicity of bacteria, disruption of the systems that regulate their generation has been an aim of drug discovery efforts. Additionally, exploiting the ability of microorganisms to generate unique biopolymers has provided for a wide range of medical and technical applications, with genome sequencing and modern molecular methods producing engineered bacteria that generate custom biomolecules.
Polysaccharides
Polysaccharides are produced by bacteria and stored internally as an energy source, or are otherwise presented on the cell surface or excreted to contribute towards the formation of a biofilm. Some bacterial species utilize polysaccharides to assist in the evasion of the host immune response by mimicry of native antigens, as they can be produced with a variety of characteristics, being charged or non-charged, homo or heteropolymers, branched or unbranched, etc.
The synthesis of polysaccharides by chemical methods is costly and difficult, limited to the production of low molecular weight molecules, and therefore microbial cell factories are necessary to produce them.
The adjustable nature of polysaccharides allows them to be tailored to a variety of purposes, for example, a high presence of hydrophilic groups on the polymer produces hydrogels that are used in drug delivery to improve the “drug-like” qualities of other compounds.
Bacterial cellulose polysaccharides, generated from monomeric D-glucose, have been explored as wound dressings and fabrics, and adjustment of the number and type of glycosidic linkages, branches, and other structural features of the monomer produces materials of differing consistency and stability, suitable for a range of applications.
Subsequent processing and incorporation with other materials can then further promote the desired characteristics, by crosslinking with citric acid or esterification with steric acid, properties such as viscoelasticity, porosity, and strength can be adjusted. These materials have the potential to act as bio-ink for 3D printers, facilitating the production of artificial cell scaffolds and tissues.
Other biopolymers
Polyphosphates are used for energy storage within bacteria, achieved by numerous energy-rich anhydride bonds, making them negatively charged. This also ensures that they interact strongly with positively charged polymers or ions, and allows them to be incorporated into hydrogels to further adjust the physicochemical properties of the gel.
Biopolymers constructed from amino acids are also produced by bacteria, also playing a role in biofilm formation, signaling, or as storage materials. Polyamides are charged molecules and can be formed into hydrogels and nano or microparticles with numerous medical and industrial applications, replacing chemically synthesized polymers.
How is spider silk used in biomedicine?
Polyesters are also produced by a range of Gram-positive and negative bacteria, though their natural function is less well understood than other biopolymers. Polyhydroxyalkanoates (PHA) are a specific group of polyesters produced by P. aeruginosa, and PHA– mutants show reduced adherence on some surfaces and weaker stress tolerance in biofilms.
It has also been suggested that PHA can act as an electron sink to enhance the survival of bacteria in anaerobic conditions, though for whatever purpose bacteria produce the biopolymer, genetic tailoring allows the mechanism to be exploited for the production of bioplastics with favorable biocompatible and biodegradable properties.
Mass production and customization
Bioplastics can be produced at the molecular or macro scale, allowing a range of objects such as nano-sized drug delivery vehicles or much larger tissue scaffolds to be produced, along with much higher volume, lower value objects, such as plastic water bottles.
Biopolymer generation is mediated by the regulatory networks of a bacterium and environmental signals at both the transcriptional and post-transcriptional levels, and it is control of these factors that allow microbial cell factories to mass-produce biopolymers.
As discussed, high molecular weight polymers of many types are only producible in good quantity by bioproduction methods, with bacteria such as P. aeruginosa generating polysaccharides 3.9 MDa in size and Komagataeibacter sucrofermentans generating over 1,000 KDa cellulose, much larger than is easily achieved by traditional chemical synthesis.
Rational reprogramming of these and other bacteria by CRISPR has allowed not only the promotion of biopolymer generation, but also the introduction of genetic switches that can utilize external signals such as light or temperature to initiate and control biopolymer production, acting as switches, timers, logic gates, and other mechanisms of control that tune the expression of biopolymers toward the desired product.
References:
- Moradali & Rehm (2020) Bacterial biopolymers: from pathogenesis to advanced materials. Nature Reviews Microbiology. https://www.nature.com/articles/s41579-019-0313-3#Sec8
- Chen & Jiang (2017) Engineering bacteria for enhanced polyhydroxyalkanoates (PHA) biosynthesis. Synthetic and Systems Biotechnology. https://pubmed.ncbi.nlm.nih.gov/29318199/
- Wilkening & Federle (2017) Evolutionary Constraints Shaping Streptococcus pyogenes-Host Interactions. Trends in Microbiology. https://pubmed.ncbi.nlm.nih.gov/28216292/
Further Reading
- All Polymers Content
- What is Polyethylene Glycol (PEG)?
Last Updated: Oct 4, 2021
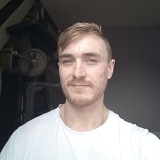
Written by
Michael Greenwood
Michael graduated from Manchester Metropolitan University with a B.Sc. in Chemistry in 2014, where he majored in organic, inorganic, physical and analytical chemistry. He is currently completing a Ph.D. on the design and production of gold nanoparticles able to act as multimodal anticancer agents, being both drug delivery platforms and radiation dose enhancers.
Source: Read Full Article